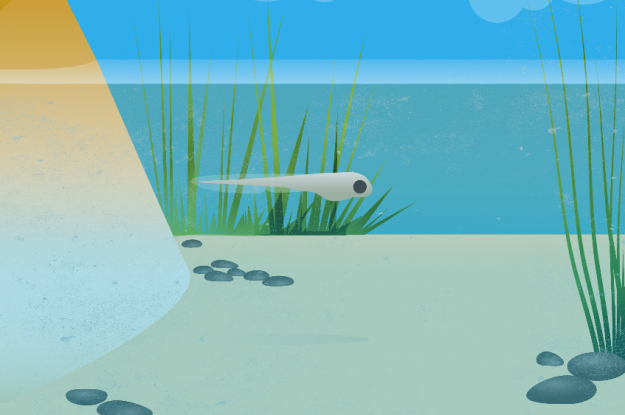
Animals on the move must adjust their physical exertion as changes in the environment or their own bodies—muscle fatigue or a strong headwind, for example—alter how efficiently they walk, swim, or fly. Scientists at the Howard Hughes Medical Institute’s Janelia Research Campus have now monitored neural activity in fish as they adapt their swimming to shifting currents, and discovered that the cells responsible for this kind of motor learning are clustered within a major mood-regulating center of the brain.
The research, published October 27, 2016, in the journal Cell, indicates that cells in the brain’s dorsal raphe nucleus (DRN) use visual clues to assess whether attempts at movement have been effective, then use that information to optimize an animal’s future actions. The finding is surprising because this part of the brain is best known for its role in controlling emotions and its potential involvement in psychiatric disorders such as major depression.
Other brain circuits may use these same cells to monitor the outcomes of more complex behaviors and adapt those behaviors accordingly, says Janelia group leader Misha Ahrens, who led the research. Learning more about how the cells work could improve understanding of the DRN’s role in emotional processing and mental illness.
Ahrens studies the neural circuits that underlie motor learning and short-term memory in zebrafish, whose small size and transparent body offer opportunities to study brain-wide activity on a cellular level. A virtual reality swim simulator lets Ahrens and his colleagues control the environment that a fish experiences while it swims, so that they can study how the fish responds as its environment changes.
Fish do not move inside the lab’s virtual reality set-up—they remain motionless, embedded in an agarose gel. But motor neurons in their tails fire as if they were swimming, and moving images projected into the simulator give the animals the sense that they are either propelling themselves forward or being swept downstream. By controlling how fast the view changes, researchers can make fish feel as if they are moving too fast or too slow. After a short swim in the simulator, fish usually rest briefly, then resume swimming at an adjusted pace.
“If you repeatedly train the fish with excessive feedback or too little feedback, they learn the appropriate motor strength and they will remember it for a while, even when they’re not swimming,” explains Takashi Kawashima, a research scientist in Ahrens’s lab.
Ahrens and Kawashima reasoned that there must be cells in the zebrafish brain that monitor changing surroundings during a swim, then retain a memory of how much progress was made until the fish swims again—this time at pace better suited to environmental conditions. There were few hints as to where those cells might be, but the team was able to search for them using new imaging technology developed by Ahrens and Janelia group leader Philipp Keller.
Although most imaging methods force users to choose between seeing fine details or viewing the big picture, in 2013 Ahrens and Keller devised a way to image the entire brain of a larval zebrafish fast enough and with sufficient resolution to observe its activity cell by cell. Kawashima used that technology to look for cells with activity patterns suggesting a role in motor learning. To retain a memory of how successful a swim had been, the scientists knew, those cells would need to fire during the resting period between swims. That memory—and thus the cells’ activity—would strengthen with repeated training. Additionally, the cells’ activity would correlate to adaptive changes during the next swim.
“We imaged the whole brain [looking for cells with these features], and a single nucleus beautifully popped up,” Kawashima says. Surprisingly, the cells that met the profile were clustered within the DRN.
“We never would have found this without whole-brain imaging, because we never would have thought to look there,” says Ahrens.
Once they had focused their attention on these DRN cells, the researchers performed additional experiments to confirm their involvement in motor learning. They found that artificially switching the cells on during the period between swims caused animals to adjust their effort during subsequent swims even more. In contrast, when they eliminated the cells or blocked their neural transmission, fish lost their ability to remember their adjustments in swim vigor.
Ahrens and Kawashima say these cells in the DRN are likely to be multifunctional. “This idea of integrating the outcomes of actions could generalize to [situations] where the action is more complicated than a swim bout, and the outcome is more complicated than visual flow,” Ahrens says. For instance, there is some evidence that the DRN is involved in animals’ willingness to actively respond to challenging situations. “In those types of cases, animals might use an internal estimate of the effectiveness of their actions to feed into decisions of whether to try or remain passive,” Ahrens says.
“We hope that this research might be a step toward explaining the more psychological functions that people have found this nucleus to be involved in,” Kawashima says.