The dynamic regulation of water channels
Membrane protein complexes
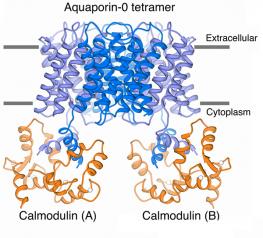
Membrane transporters involved in nutrient uptake
Sugar uptake
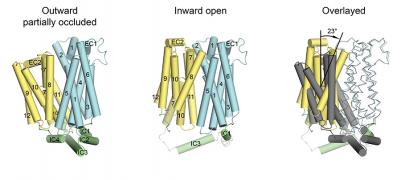
Nitrogen uptake
Nitrate is the preferred nitrogen source for plants on which all higher forms of life ultimately depend. Plants and microorganisms evolved to efficiently assimilate nitrate. Despite decades of effort no structure was available for any nitrate transport protein and the mechanism by which nitrate is transported remained largely obscure until our study was published. We reported the structure of a bacterial nitrate/nitrite transport protein, NarK, from Escherichia coli, with and without substrate. The structures revealed a positively charged substrate-translocation pathway lacking protonatable residues, suggesting that NarK functions as a nitrate/nitrite exchanger and that H+s are unlikely to be co-transported. Conserved arginine residues form the substrate-binding pocket, which is formed by association of helices from the two halves of NarK. Key residues that are important for substrate recognition and transport were identified and related to extensive mutagenesis and functional studies. We proposed that NarK exchanges nitrate for nitrite by a rocker-switch mechanism facilitated by inter-domain H-bond networks.
Relevant papers:
1. Gonen T., Sliz P., Cheng Y., Kistler J. and Walz T. (2004) Aquaporin-0 membrane junctions reveal the structure of a closed water pore. Nature. 429 : 193 – 197.
2. Gonen T., Cheng Y., Kistler J. and Walz T. (2004) Aquaporin-0 membrane junctions form upon proteolytic cleavage. Journal of Molecular Biology 342 : 1337 - 1345.
3. Gonen T., Cheng Y., Sliz P., Hiroaki Y., Fujiyoshi Y., Harrison SC., Walz T (2005) Lipid–protein interactions in double layered two dimensional crystals of AQP0. Nature 438: 633 - 638.
4. Reichow L.S. and Gonen T*. (2008) Non-canonical binding of calmodulin to aquaporin-0: implications for channel regulation. Structure. 16: 1389 – 1398.
5. Gold MG, Reichow SL., O’Neill SE, Weisbrod CR, Langeberg LK, Bruce JE, Gonen T* and Scott JD*. (2012) AKAP2 anchors PKA with aquaporin-0 to support ocular lens transparency. EMBO Molecular Medicine 4: 15-26.
6. Reichow S.L, Clemens D.M., Freites J.A., Németh-Cahalan K.L., Heyden M., Tobias D.J., Hall J.E*. and Gonen T*. (2013) Allosteric mechanism of water channel gating by Ca2+–calmodulin. Nature Structural and Molecular Biology – 20 (9): 1085 - 1092.
7. Smith FD., Reichow LS., Esseltine JL., Shi D., Langeberg LK., Scott J* and Gonen T* (2013). Intrinsic disorder within an AKAP-Protein kinase A complex guides local substrate phosphorylation. eLife 2:e01319.
Relevant Reviews:
1. Gonen T*. and Walz T* (2006) The structure of Aquaporins. Quarterly Reviews in Biophysics 39 : 361 -396.
2. Engel, A., Fujiyoshi, Y., Gonen, T. and Walz, T. (2008) Junction forming aquaporins. Current Opinion in Structural Biology. 18 : 229 - 235.
3. Andrews, S.A., Reichow, L.S. and Gonen T*. (2008) Electron crystallography of aquaporins. IUBMB Life 60: 430 – 436.
4. Reichow, S.L. and Gonen T*. (2009) Lipid-protein interactions probed by electron crystallography. Current Opinion in Structural Biology. 19: 560 - 565
5. Gold M. Gonen T and Scott J. (2013) Local cAMP signaling in disease at a glance. Journal of Cell Science. 126: 4537 - 4543.
Relevant papers
1. Zheng H., Taraska J., Merz A. and Gonen T*. (2010) The prototypical H+/galactose symporter GalP assembles into functional trimers. Journal of Molecular Biology. 396 : 593 – 601.
2. Wisedchaisri G., Dranow D.M., Lie T.J., Bonanno J.B., Patskovsky Y., Ozyurt S.A., Michael Sauder J.M., Almo S.C., Wasserman S.R., Burley S.K., Leigh J.A. and Gonen T*. (2010) Structural underpinnings of nitrogen regulation by the prototypical nitrogen-responsive transcriptional factor NrpR. Structure 18 1512 - 1521.
3. Zheng H, Wisedchaisri W and Gonen T*. (2013) Crystal structure of a nitrate/nitrite exchanger. Nature – 497: 647-651.
4. Wisedchaisri G., Park MS., Iadanza MG., Zheng H. and Gonen T*. (2014) Proton-coupled sugar transport in the prototypical major facilitator superfamily protein XylE. Nature Communications – (5)4521: 1 - 11.